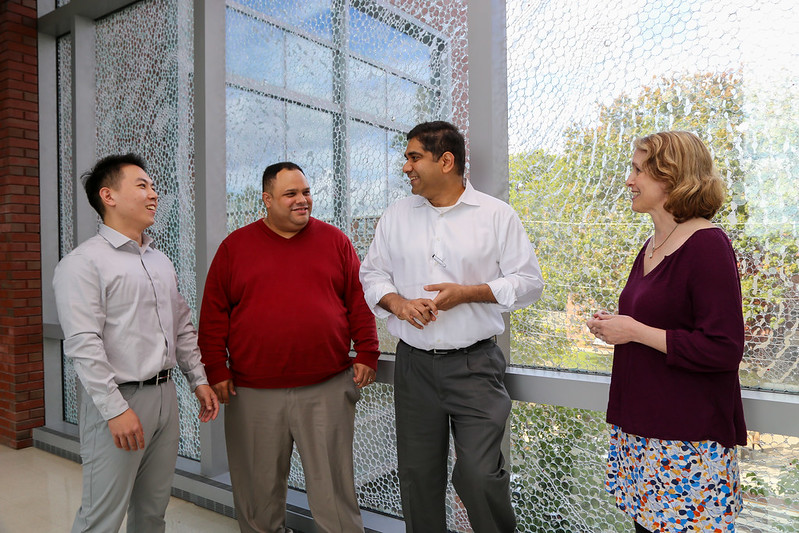
For nearly a decade, Diane Hoffman-Kim's lab has made cortical spheroids – basically working mini-brains.
Hoffman-Kim, an associate professor of neuroscience and engineering and a faculty affiliate of the Robert J. and Nancy D. Carney Institute for Brain Science, and her tissue engineering teams have used these three-dimensional cell cultures to study human brain injury and stroke. Currently, the team is using them to study glioblastoma, a deadly brain cancer. Because of their versatility and novelty, cortical spheroids have captured the imagination of scientists and the public – witness this story in WIRED magazine.
In new work, published August 19 in PLOS One, Hoffman-Kim and a team of Brown biomedical engineers used for the first time cortical spheroids to test the effects of compression injuries, which cause brain damage that takes minutes, days or months to surface.
Compression injuries are often caused by stroke or tumors but can also be caused by trauma. A ski accident, car crash or soccer collision can, over time, cause blood to pool or tissue to swell and create pressure on brain tissue. Gone untreated, compression injuries can cause chronic inflammation, kill brain cells and even cause death.
Hoffman-Kim has used cortical spheroids to study brain injuries before – but not compression injuries. Along with her team, she was intrigued because these injuries are common and because they pose a research challenge. Could they mimic in the lab the conditions of slow, steady pressure on brain cells?
Hoffman-Kim and Haneesh Kesari, Ph.D., an associate professor in Brown’s School of Engineering, are partners on a long-term research project, funded by the U.S. Office of Naval Research, to study brain injuries for the military.
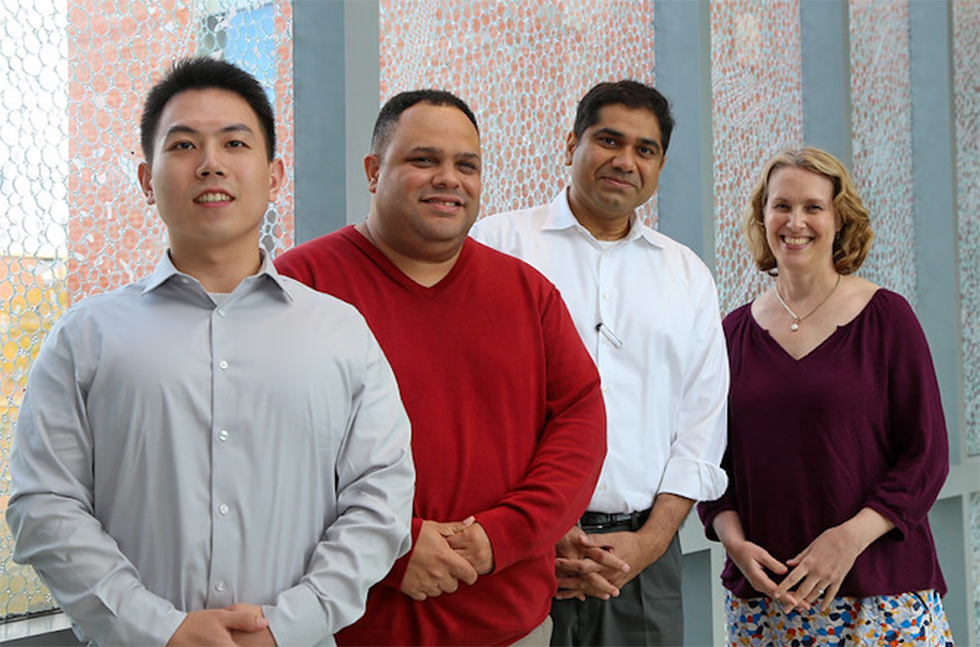
They tossed around ideas with Rafael González-Cruz, a postdoctoral scientist in Brown’s Department of Neuroscience who also conducts work with Kesari. González-Cruz is part of Carney’s Advancing Research Careers, or ARC, program. ARC provides professional development and mentorship to early career women and people historically excluded from science to help them launch the next stage of their research career.
To make mini-brains, González-Cruz uses a centrifuge, a lab instrument that rotates at fast speeds to create a huge amount of force – enough to separate cell types. Kesari wondered if a centrifuge could also be used to create the kind of pressure that comes with a compression injury.
Why not? González-Cruz said.
They reached out to Yang Wan, a Ph.D. student in the School of Engineering. Wan helped González-Cruz and Kesari work out an experimental design that would allow them to test and measure what happens to mini-brains when different levels of sustained force are applied.
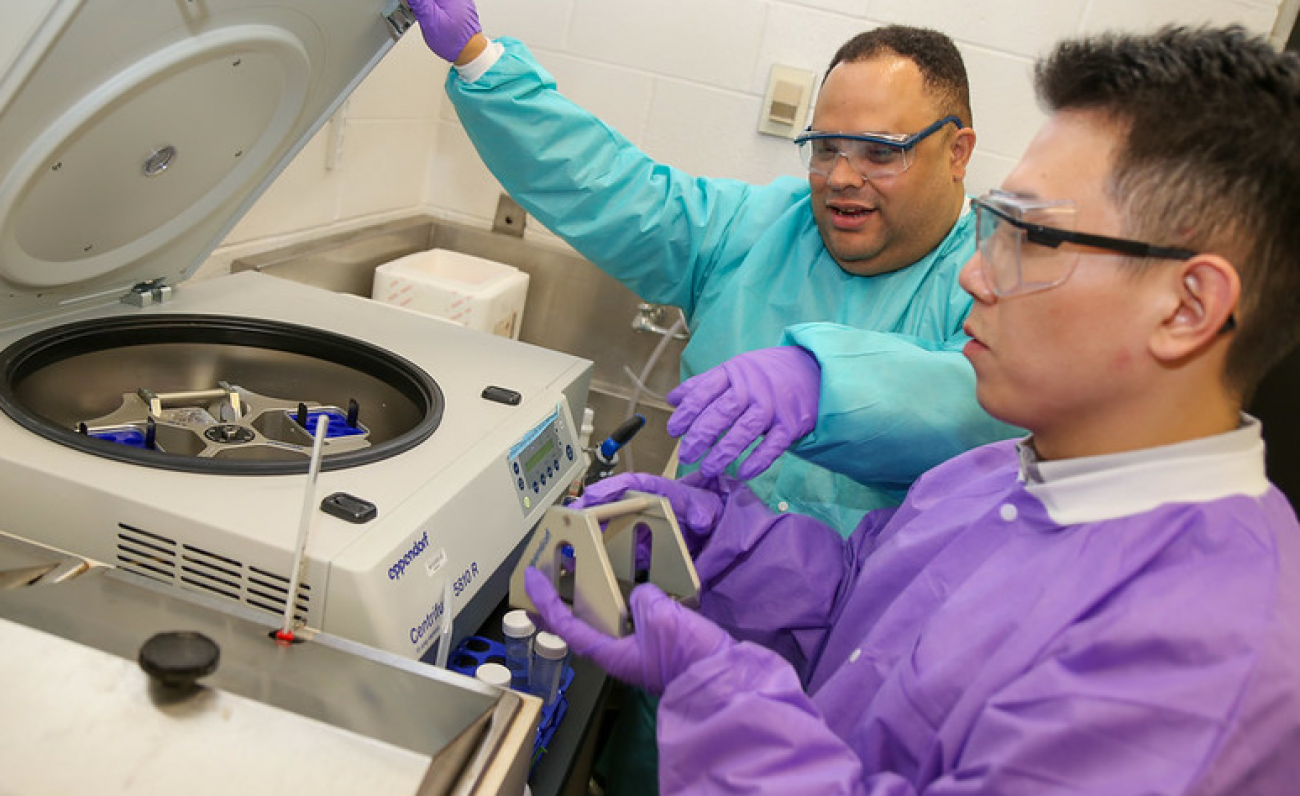
They ran batches of mini-brains in a centrifuge for two minutes – a long period of time compared to a split-second impact. Wan calculated four specific speeds, along with a control, so the team could test all the centrifuge speeds uniformly.
After batches of brains were spun, researchers counted dead brain cells and studied injured ones. They repeated their assessment two hours later, then eight hours later, then 24 hours later. This 24-hour period is important, they note in their paper, as that’s the window when medical intervention would be most effective.
This study design allowed the team to measure, with precision, the cell damage not only caused by different amounts of force but the amount of damage that unfolds over longer periods of time – the slow-burn effect that happens with compression injuries.
Researchers found that a minimum speed was required for the centrifuge to produce enough force to cause significant cell death and DNA damage, and that the amount of cell death and damage increased over time.
What’s important about this finding, Kesari and Hoffman-Kim say, is that researchers were able to quantify the cell damage that occurs after different levels of force were applied and to study that impact across different time scales – information critical for understanding how it might be possible to prevent, diagnose and treat compression injuries.
“Mini-brains have so many research benefits,” Hoffman-Kim said. “We’re humbled that something this much smaller than the brain can actually mimic diseases – and now injuries.”
The social relevance, and scientific elegance, made this research project particularly fulfilling, Kesari said.
“These results can have a real impact on people and help keep them safe and healthy,” Kesari said. “I don’t often get to do work like this. This is the kind of interdisciplinary science with broad societal impact that I dreamed about doing when I was growing up.”
The holy grail, the research team says, will be someday to have the ability to test someone’s blood after an injury and gauge the level of proteins associated with injury. González-Cruz is already studying these proteins.
“This work has resulted in a reliable, precise platform to study brain injury,” González-Cruz said. “With our model, researchers can study the relationships between injury severity and cellular events that lead not only to cell death after a traumatic event, but also inflammation and neurodegeneration that can occur over time. And we can do it in a lab in a dish, in a highly reproducible way that’s critical for good science.”
González-Cruz and Wan are the lead authors of the PLOS One article. Hoffman-Kim and Kesari are senior authors.
The research team also included Amina Burgess, Dominick Calvao, and Francesca Vecchio with Brown’s Institute for Biology, Engineering and Medicine and William Renken from Brown’s Department of Neuroscience, as well as Christian Franck, with the Center for Traumatic Brain Injury and the Department of Mechanical Engineering at the University of Wisconsin-Madison. The team is part of the PANTHER Program, a national research collaborative that brings university, industry and government experts together to better detect, prevent and treat traumatic brain injuries.
The work was funded by the Office of Naval Research under award number N000142112044. González-Cruz received support through National Institutes of Health Research Education Grant R25NS124530 to the Carney Institute and got support under National Institutes of Health Institutional Research Training Grant T32HL134625 through Brown’s Division of Biology and Medicine.